Public vs. Private Keys: Your Guide to Online Security and Privacy
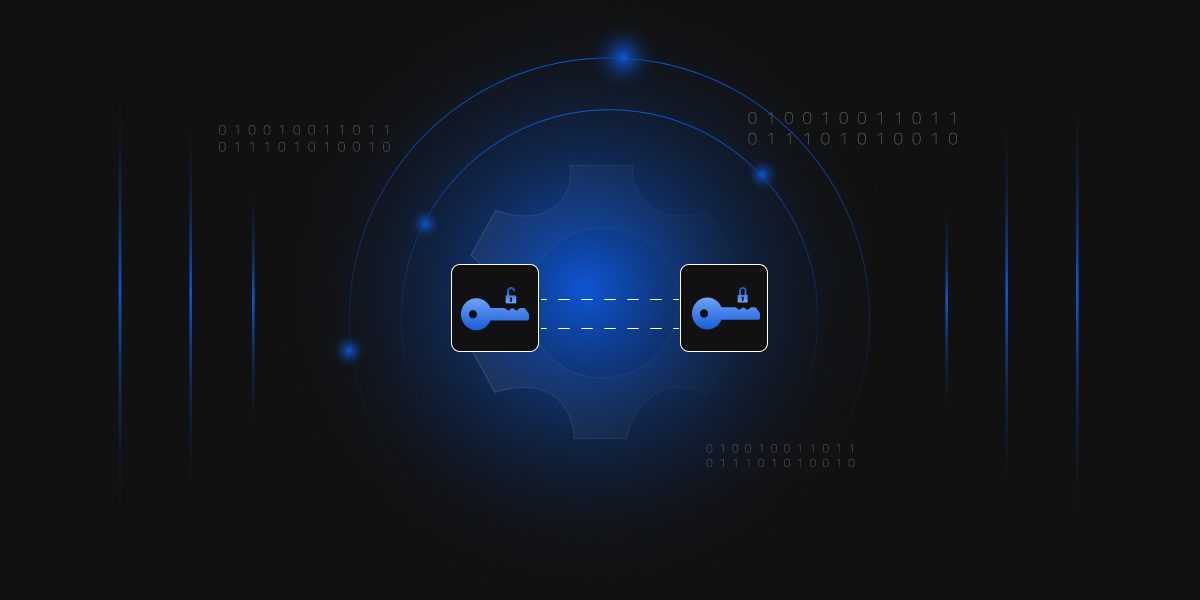
Imagine you send a message to a person on the other side of the world. You lock the message in a box with a key, and then send it across continents, hoping no one interrupts it. But there’s a problem—if anyone, anywhere, manages to get a copy of that key, they can unlock the box and read everything inside.
This was the unfortunate state of digital communication in the early days. Before the internet became the vast, interconnected web we know today, people relied on a single shared key to encrypt and decrypt messages—a method known as symmetric cryptography, where the same key is used for both encryption and decryption, such as in algorithms like AES (Advanced Encryption Standard) or DES (Data Encryption Standard).
It worked well enough in controlled environments, but as communication expanded globally, symmetric cryptography faced a major challenge: securely distributing the encryption key. There was always the risk of eavesdropping, where attackers could intercept the key during transmission. And as more people connected, the system became harder to manage—scaling securely across countless users was nearly impossible. So the question arose: how could you safely share a key with someone halfway across the globe without anyone intercepting it?
As the popularity of the internet increased, sensitive information like bank details, corporate secrets, government intelligence, and private conversations began moving through exposed digital networks. This exposed the limitations of symmetric cryptography.
Then came a revolutionary idea that would save the future of cybersecurity: public and private key cryptography, also known as asymmetric cryptography. Instead of relying on a single secret key shared between sender and receiver, this new method uses two keys: one public, one private. The public key could be shared openly with anyone, while the private key remained safely guarded. Messages encrypted with the public key could only be decrypted with the corresponding private key, and vice versa.
Suddenly, it became possible to send confidential information over insecure networks without ever needing to exchange secret keys in advance. Techniques like RSA and ECC made this possible—mathematically complex systems that form the backbone of secure communication today.
Now, let’s look at how public and private keys work together.
Public and private keys work like a digital lock-and-key system, ensuring that online communication remains private, secure and trustworthy. They work in two main ways:
Category | Aspect | Public Key | Private Key |
Components | RSA Elements | Modulus (n), Public exponent (e) | Modulus (n), Private exponent (d) |
ECC Elements | High Curve name, Public point (Q = d × G) | Curve name, Private scalar (d) | |
Visibility | Visibility | Shared publicly | Kept secret |
Distribution | Freely distributed (e.g., with TLS certificates) | Never shared; kept secure | |
Usage | Purpose | Encrypt data, verify digital signatures | Decrypt data, create digital signatures |
Who Uses It | Anyone (recipients, verifiers) | Only the owner | |
Examples | Email encryption, website certificate validation | Digital signing, secure authentication | |
Storage | File Formats | .crt, .cer, .pem | .key, .pem, .pfx |
Typical Storage Location | Public repositories, certificates | Kept in secure storage (HSMs, encrypted files) | |
Security | Role in Security | Establishes trust and enables secure communication | Enables confidentiality and authentication |
If Exposed | Trust may be reduced (e.g., impersonation) | Severe risk — allows full compromise of encrypted/signed content | |
Ownership & Control | Associated with identity (e.g., domain, person) | Controlled exclusively by the key owner |
When you visit a secure website like https://example.com:
As part of its SSL/TLS certificate, the website sends you its public key. Using this key, your browser and the website perform a process called the TLS handshake to establish a secure communication channel. During this handshake, a session key is created using public key cryptography. This session key is then used to encrypt and decrypt all data exchanged between the browser and the website, ensuring fast and secure communication.
While the website’s public key is used during the handshake to establish the session key, the actual data (like passwords) is encrypted using this session key, not the public key itself. Only the website’s private key can decrypt the session key securely, ensuring that your sensitive data can only be read by the real site.
The ownership of your crypto wallet is verified through your private key, which is used to sign transactions. Your public key, on the other hand, is used to derive your wallet address, which is shared publicly to receive funds.
In the case of Bitcoin, the wallet address is a hashed version of your public key, and it’s the address others use to send you cryptocurrency. If someone knows your public key (or wallet address), they can send you crypto—but only your private key can sign and authorize transactions to spend that crypto, ensuring that only you can control the funds in your wallet.
With PGP (Pretty Good Privacy) or its open-source implementation, GPG (GNU Privacy Guard), you give your public key to friends, allowing them to encrypt emails sent to you. To encrypt the message, PGP/GPG first uses symmetric encryption to encrypt the actual message, ensuring efficient encryption of larger content. Then, it encrypts the session key used for symmetric encryption with your public key, leveraging asymmetric encryption for secure key exchange.
When you receive the email, you use your private key to decrypt the session key, and with the session key, the message itself is decrypted. This ensures that only you, with your private key, can read the encrypted email.
SSH (Secure Shell) typically uses public key authentication with a challenge-response mechanism to securely authenticate users. In this model, your private key is stored on your computer, while the public key is stored on the server.
When you attempt to connect, the server generates a random challenge (usually a large number or string), which is sent to your client. The client then uses the private key to sign the challenge. This signed response is sent back to the server. The server, having the public key, can verify the signature. If the server successfully verifies the response, it grants access to the user.
This challenge-response model works as a secure proof of possession: even if an attacker intercepts the challenge, they cannot respond without having access to the private key. This method is significantly stronger than password-based authentication because the private key never leaves your device, reducing the risk of interception. Moreover, the challenge-response mechanism ensures that only someone with access to the correct private key can authenticate, making it highly resistant to brute force or phishing attacks.
When developers release software updates, they sign the updates using a private key. This signature doesn’t encrypt the software itself; instead, it ensures the integrity and authenticity of the update, allowing you to verify that the software has not been tampered with and is indeed from the legitimate developer.
Your device uses the developer’s public key to verify the signature against the update, checking that it matches the original software. If the signature is valid, you can be confident that the update is authentic and has not been altered during transmission.
Common code-signing algorithms like RSA or ECDSA are used to create and verify these digital signatures, ensuring the security of the software distribution process.
In public-key cryptography security is important because compromise can lead to data breaches, impersonation, and fraud. Here’s a breakdown of key security considerations:
The private key is the cornerstone of asymmetric cryptography—if it’s compromised, an attacker can decrypt sensitive data, forge signatures, or impersonate the legitimate user. Proper protection is therefore critical.
Best Practices for Protection:While public keys are meant to be shared, verifying who they belong to is crucial. Without proper verification, attackers can perform Man-in-the-Middle (MitM) attacks by substituting their own keys.
Methods for Verifying Public Key Authenticity:Example: In HTTPS (TLS), a browser trusts that it’s talking to bank.com because the site’s certificate is signed by a known CA.
Example: Alice verifies Bob’s key and signs it. Carol may trust Bob’s key because she trusts Alice.
Example: Mobile apps often use certificate pinning to prevent accepting spoofed certificates.
Example: During SSH setup, users may compare key fingerprints via a secure, trusted communication channel.
At Encryption Consulting, we specialize in designing, implementing, and managing secure Public Key Infrastructure (PKI) solutions tailored to your organization’s needs.
Whether you’re aiming to strengthen encryption, enable secure digital identities, or ensure compliance with industry standards, our PKI services provide the foundation for secure communication and digital trust.
Our offerings include:
Our expertise ensures that your use of public and private keys is not only technically sound but also operationally resilient, protecting your data, applications, and users in today’s threat landscape.
Public and private key cryptography has become the backbone of modern digital security. It solves the core problem of trust in open networks—enabling secure communication, identity verification, and data integrity without the need to secretly exchange keys. From browsing secure websites and sending encrypted emails to managing cryptocurrency wallets and verifying software updates, public-private key pairs are quietly working behind the scenes to protect our digital lives.
By understanding how these keys work and why protecting them is important, individuals and organizations can take informed steps to strengthen their cybersecurity. As online threats continue to grow, Public Key Infrastructure (PKI) remains one of the most powerful tools we have to ensure privacy, security, and authenticity in an increasingly connected world.
Looking ahead, with the emergence of quantum computing, traditional public key algorithms may become vulnerable. Organizations should begin exploring post-quantum cryptography to prepare for the next era of cryptographic security.